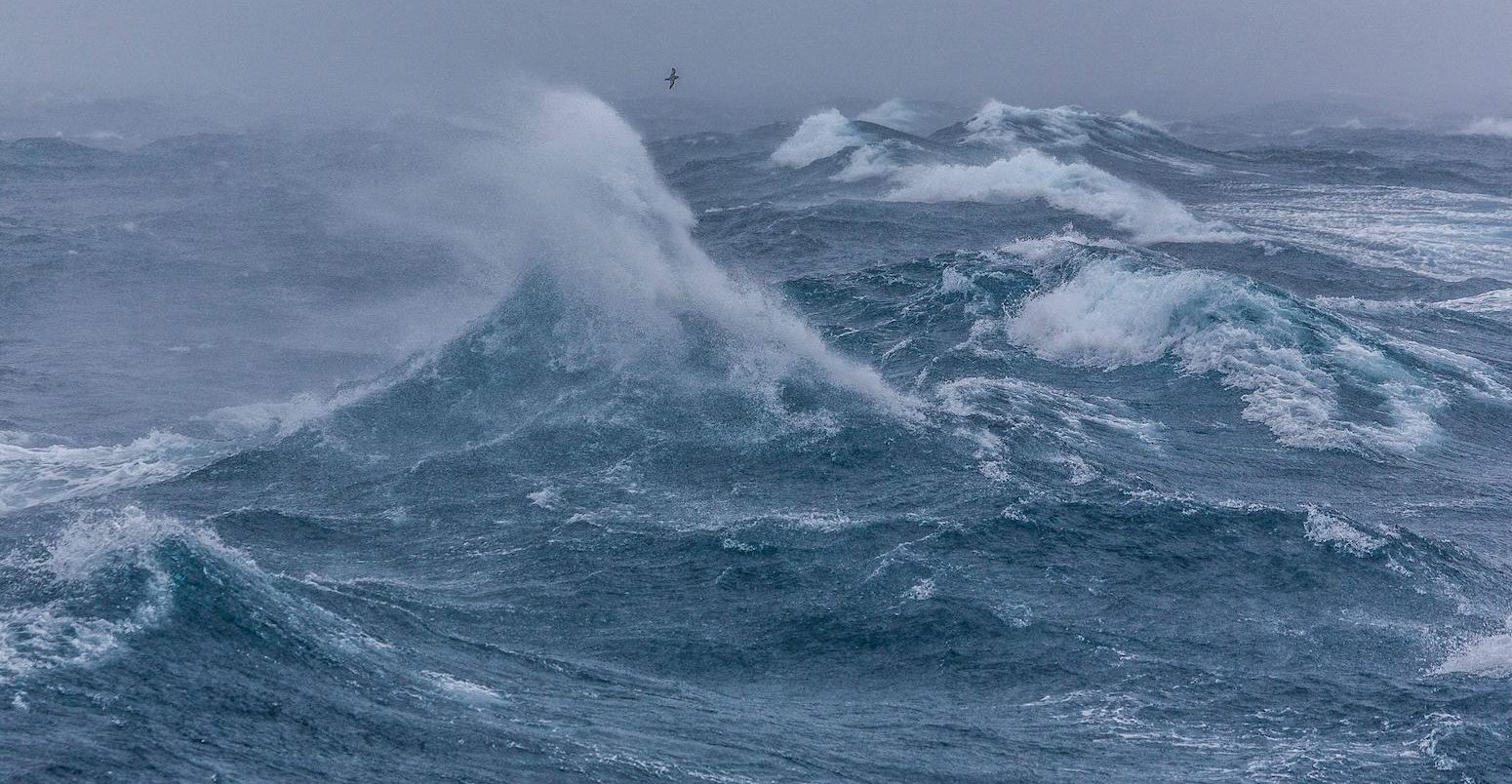
Guest post: Why the southern hemisphere is stormier than the northern

Prof Tiffany Shaw
12.12.22
Prof Tiffany Shaw
12.12.2022 | 3:00pmThe southern hemisphere is a very stormy place. The winds at different degrees of latitude are described as the “roaring forties”, “furious fifties” and “screaming sixties”. Ocean wave heights can reach an astounding 78 feet (24 metres).
It is well-known that the northern hemisphere does not have anything comparable to the extreme storms, winds and waves of the south. Why?
In a new study, published in the Proceedings of the National Academy of Sciences, my co-authors and I unravel why the southern hemisphere is stormier than its northern neighbour.
Combining multiple lines of evidence from observations, theory and climate models, our findings point to the fundamental role of the global ocean “conveyor belt” and the large mountain ranges in the northern hemisphere.
We also show that the southern hemisphere is getting even stormier over time, whereas the north is not. This is consistent with what climate models simulate for a warming world.
These changes are important because we know stronger storminess can result in more high-impact events, such as extreme winds, temperatures and rainfall.
A tale of two hemispheres
For a long time, most weather observations on Earth were taken on land. This gave scientists a clear picture of storms in the northern hemisphere. However, for the southern hemisphere, which is around 20% land, it was not until the advent of satellite-based observations in the late 1970s that we obtained a clear picture of storms there.
Thanks to decades of observations since the beginning of the satellite era, we know the southern hemisphere is about 24% stormier than the northern hemisphere.
This is illustrated by the maps below, which show the observed annual average storminess across the southern hemisphere (top), northern hemisphere (middle) and the difference between the two (bottom) for 1980-2018. (Note that the south pole is at the top for the first map and the comparison in the last map.)
The maps show the levels of storminess are consistently high across the Southern Ocean in the southern hemisphere and concentrated in the Pacific and Atlantic oceans in the northern (orange shading). The difference map shows the southern hemisphere is stormier than the northern across most latitudes (orange shading).
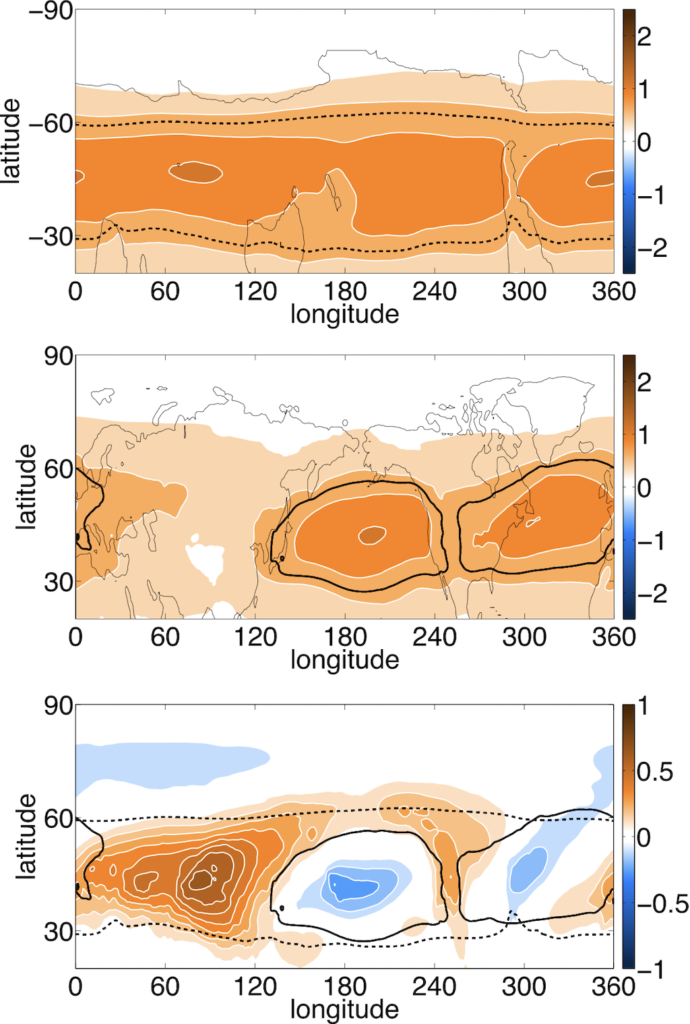
Energy difference
Despite a number of different theories, no one had provided a clear explanation for this difference in storminess between the two hemispheres.
It may seem to be a daunting task to be able to figure out why. How can one understand a system as complex as the atmosphere, which spans thousands of kilometres? We cannot put the Earth in a jar and study it. Yet this is exactly the job of scientists studying the physics of climate. We bring to bear the laws of physics and use them to understand Earth’s atmosphere and climate.
The most famous example of this approach is the pioneering work of Dr Syukuro Manabe who was jointly awarded the Nobel prize of physics in 2021 for “reliably predicting global warming”. His prediction was built on physics-based models of Earth’s climate – starting from the simplest 1D model of temperature, working up to a full 3D model. He examined the response of the climate to increasing atmospheric CO2 levels across the models of different physical complexity and traced the emergent signals to the underlying physics.
In order to unravel the case of the stormier southern hemisphere, we bring together multiple lines of evidence, including from a physics-based climate model. As a first step, we examine observations from the perspective of how energy is distributed across the Earth.
Because the Earth is a sphere, it does not receive solar radiation from the sun evenly across its surface. Most energy is received and absorbed at the equator, where the sun’s rays hit the surface more directly. In comparison, less energy is received at the poles, where the rays strike at a steep angle.
Decades of research show storms derive their strength from this energy difference. In essence, they convert “static” energy stored in this difference into “kinetic” energy of motion. This conversion occurs through a process called “baroclinic instability”.
This perspective suggests incoming sunlight cannot account for the stormier southern hemisphere because the two hemispheres receive the same amount. Instead, our observational analysis suggests the different strength of storminess in the south versus the north is likely related to two different factors.
First, the transport of energy by the ocean, which is often described as a “conveyor belt”. Water sinks near the Arctic, travels along the bottom of the ocean, rises up around Antarctica and flows back north along the equator – bringing energy with it. The net effect is that energy is moved away from Antarctica and toward the Arctic. This leaves a larger energy contrast between the equator and the pole in the south than in the north, which contributes to a stormier southern hemisphere.
The second factor is the large mountains in the northern hemisphere, which – as Manabe’s early work had hinted – suppress storms. Air flow over large mountain ranges create stationary highs and lows that leave less energy available for storms.
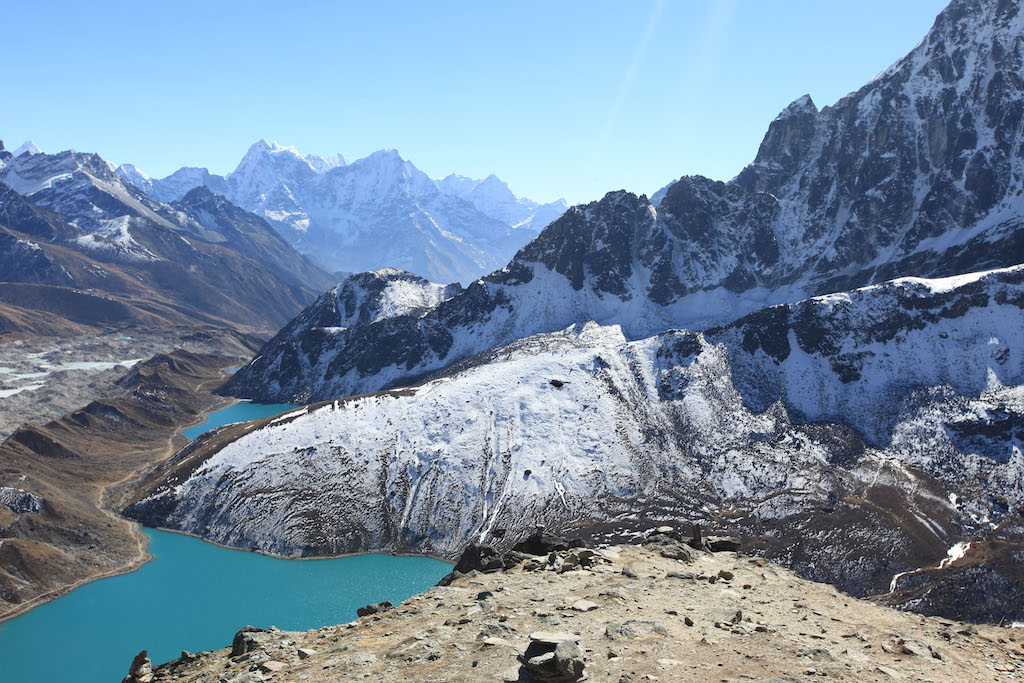
However, analysing observed data alone cannot confirm these causes because too many factors are operating and interacting all at once. Furthermore, we cannot remove individual causes in order to test their importance.
For this, we need to use climate models to examine how storms change when different factors are removed.
When we flattened the Earth’s mountains in the model simulations, the difference of storminess between the hemispheres decreased by half. When we removed the ocean conveyor belt, the other half of the difference of storminess disappeared. Thus, we had revealed the first concrete explanation for the stormier southern hemisphere.
Stronger or weaker storminess?
Since storminess is associated with significant societal impacts – such as extreme wind, temperature and rain events – an important question for us to answer is whether storminess will be stronger or weaker in the future.
A key tool for preparing society for the impacts of climate change is providing projections from climate models. The most recent research suggests that, across the year on average, the southern hemisphere will get stormier on average by the end of the century.
In contrast, changes in annual-mean storminess in the northern hemisphere are expected to be muted. This is in part because of competing seasonal influences between warming of the tropics, which would make storminess stronger, and rapid warming in the Arctic, which would make storminess weaker.
Yet the climate is changing here and now. When we examine changes over the last several decades, we find the southern hemisphere is getting even stormier on average across the year and the northern hemisphere change is negligible – consistent with climate model forecasts for the same time period.
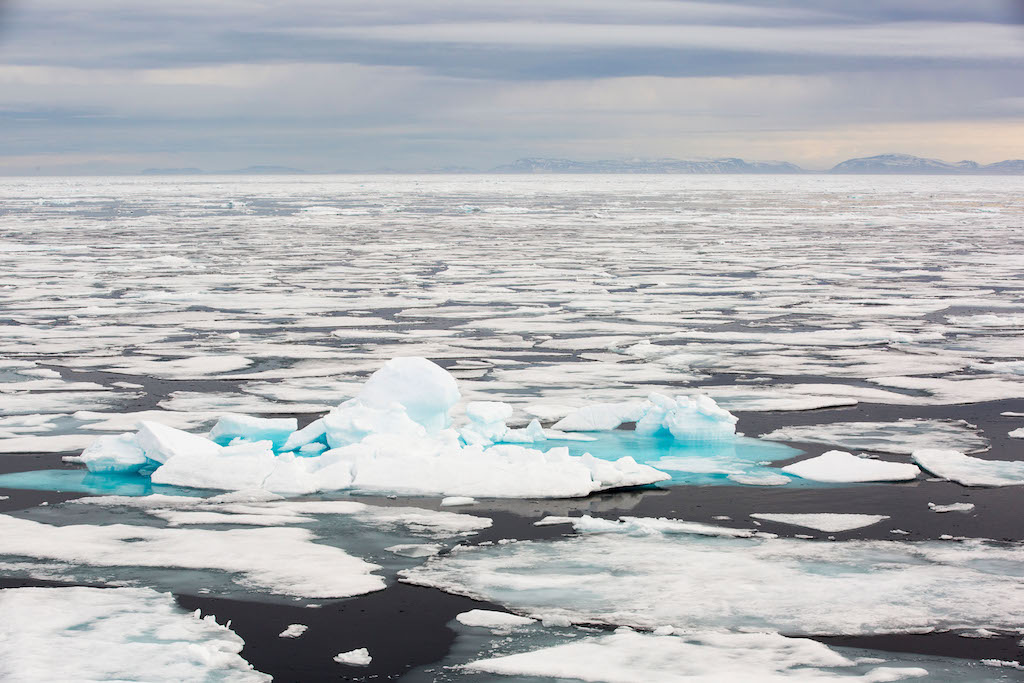
While the models underestimate the signal, they are pointing to the changes occurring for the same physical reasons. Namely, the ocean changes are increasing storminess because warm water is being moved equatorward and colder water is being brought to the surface around Antarctica to replace it, which leads to a stronger equator-to-pole contrast.
In the northern hemisphere, the ocean changes are offset by the loss of sea ice and snow, which leads to more absorption of sunlight in the Arctic and a weaker equator-to-pole contrast.
The stakes are high for getting the answer right. It is important for future work to determine why models are underestimating the signal in observations, but it is just as important to get the right answer for the right physical reasons.
Shaw, T. et al. (2022) Stormier southern hemisphere induced by topography and ocean circulation, Proceedings of the National Academy of Sciences, doi:10.1073/pnas.2123512119