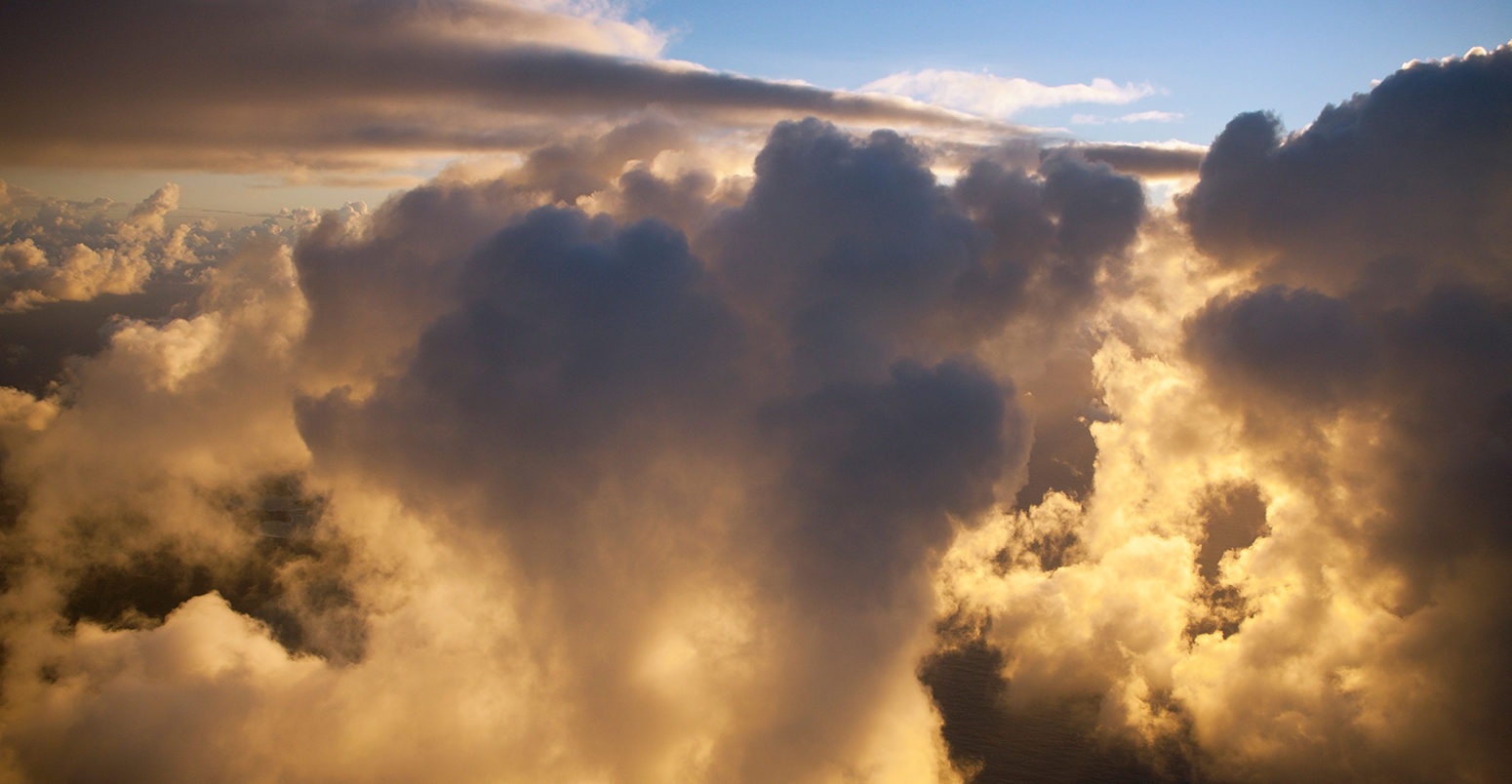
Guest post: Why clouds hold the key to better climate models

Prof Ellie Highwood
01.18.18
Prof Ellie Highwood
18.01.2018 | 8:00amProf Ellie Highwood is professor of climate physics in the Department of Meteorology at the University of Reading and president of the Royal Meteorology Society.
As a climate modeller, I find clouds both breathtakingly beautiful and infernally infuriating.
The never-ending and continuous changing nature of clouds has given rise to beautiful poetry, hours of cloud-spotting fun and decades of challenges to climate modellers.
Clouds in the present-day climate system cover approximately two-thirds of the globe. Tropical rainfall belts and mid-latitude storm tracks are particularly cloudy, whilst the subtropical oceans and continental desert regions see relatively clear skies.
These distributions are predominantly down to the large-scale circulation patterns and basic thermodynamics of moist air. However, a cloud’s own effect on climate is very sensitive to its properties.
Low, bright clouds have a largely cooling influence because they reflect sunlight away from the Earth’s surface. High, thin clouds composed of ice crystals are more or less transparent to sunlight, but do absorb outgoing infrared radiation, re-emitting a small fraction and, thereby, warming the planet. What makes things even more complicated is that, in most places, there are multiple layers of cloud at any one time.
Overall, in today’s climate, the cooling effect of clouds outweighs their warming impact.
Clouds are fickle in both space and time – appearing, changing and disappearing on timescales of minutes. This means accurately representing them in models is one of the biggest challenges facing climate scientists and the key to even more accurate projections.
Challenging clouds
One of the reasons that clouds are such a source of frustration to climate modellers is that the processes affecting how clouds form, grow and diminish happen on a vast range of scales – from sub-micrometer cloud processes to cloud systems of thousands of kilometres in size.
Cloud microphysics modellers attempt to understand and simulate the birth and growth of water droplets and ice crystals. To achieve this they must model the dynamics and thermodynamics of individual cloud droplets.
Laboratory studies can activate individual cloud condensation nuclei – in other words, coercing specks of dust into acting as condensation sites for enough water vapour so that they grow big enough to become fully-fledged cloud droplets. On a slightly larger scale, we use models with small overall domains, but very large numbers of calculation points to model the interaction of turbulence with cloud droplets. We call these models “Large Eddy Simulations” because they can represent the interaction of turbulent swirls with cloud layers, or “Cloud Resolving Models” (CRMs) because they can represent the physics of an individual cumulonimbus cloud.
We can do these high resolution calculations over larger and larger spaces thanks to high-performance computing, but still can only run for a few days over an area of a few square kilometers.
But none of the processes involved on this scale – which are ultimately responsible for determining cloud water (or ice) content, thickness and lifetime – can currently be represented explicitly in climate models that need to simulate the whole globe for extended periods of time.
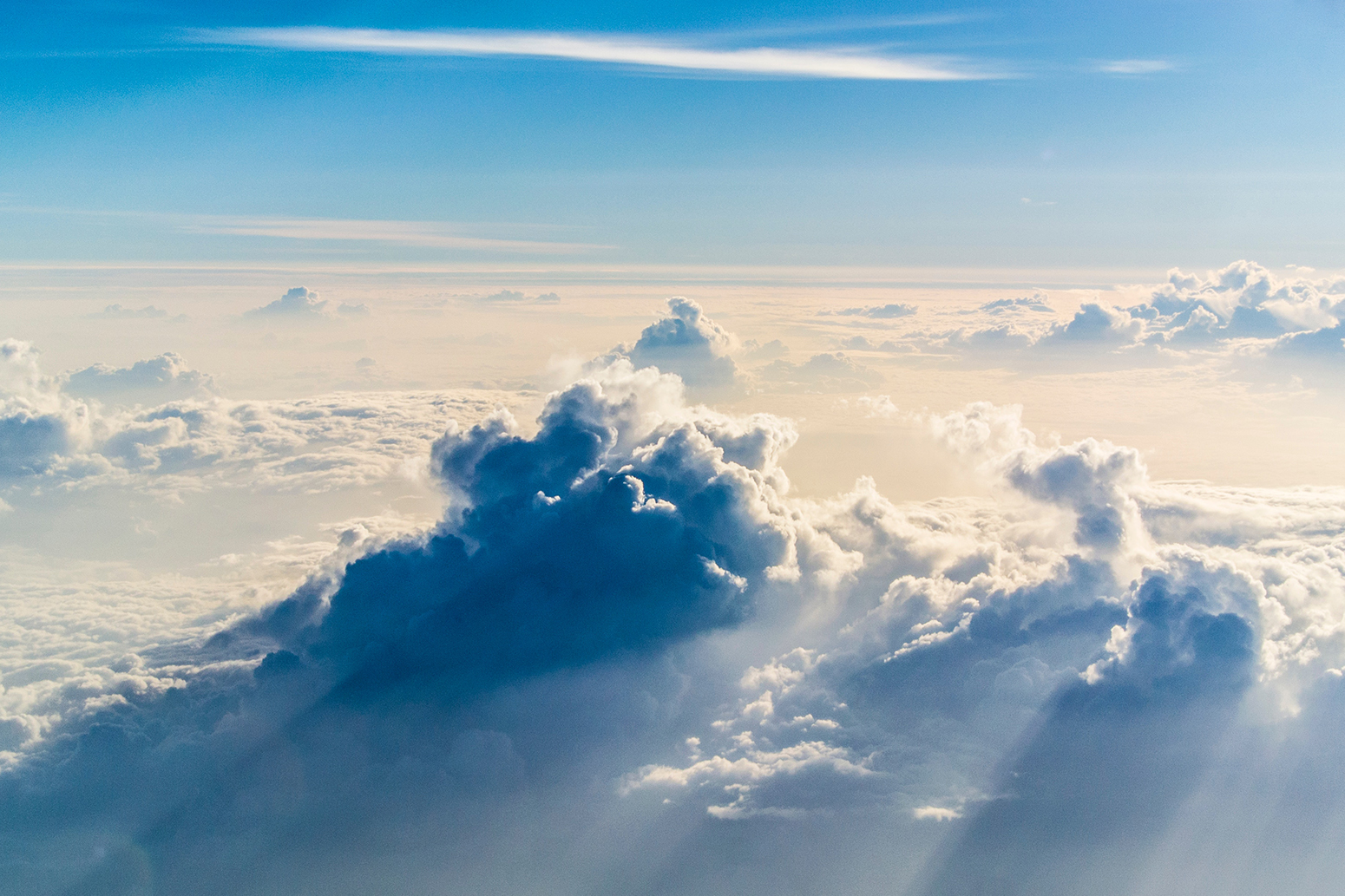
Clouds, Goa. Photo by Kaushik Panchal on Unsplash.
Model advances
Despite the difficulty of translating the complexity of clouds at small scales into simplified, large-scale models, scientists have been making advances.
In particular, some climate models have been run for short times at much higher resolution such that cumulus cloud systems can be represented across the globe. These have a better ability to couple cloud systems to atmospheric dynamics, but still have to approximate the effects of individual clouds and turbulence.
The results show that the way we make these approximations makes a real difference.
In an alternative approach, some models have also been developed that embed a full CRM within each individual cell of a climate model. These “super-parameterisations” have been shown to improve some aspects of climate model simulations. For example, they tend to correctly simulate the peak in land convection in tropical regions, which brings rainfall in the late afternoon rather than at midday – which has been a common problem in climate models with more simple representation.
The first studies with these models have also shown improvements to simulating rainfall patterns that cover large swaths of the Earth’s surface. One of these is a long-standing issue with the Inter-Tropical Convergence Zone (ITCZ), a huge belt of low pressure that encircles the Earth near the equator. Many global climate models simulate a “double ITCZ”, which brings too much rainfall to the southern hemisphere tropics. Another issue has been accurately representing the frequency and length of El Niño-Southern Oscillation (ENSO) cycles.
Improved tropical variability in climate and weather in these models also turns out to be important for accurate simulation of how the Asian monsoon develops.
Whilst we still need to understand why these super-parameterised models do better, it is thought that it might be due to the improved representation of clouds and convection doing a better job of moistening and heating the tropical atmosphere so that the deep, towering storm systems are more likely to form.
Unfortunately, though, these models still have some limitations in representing low-altitude cloud processes, and research and development is ongoing.
Observations
We also have the challenge of needing high resolution and high quality observations to evaluate our cloud models against.
Some of these can be directly provided by aircraft measurements from within clouds themselves – on relatively small scales – or through the increasing number of satellite observations of cloud properties, such as altitude, coverage and droplet size. Remote sensing data, such as LIDAR, is increasingly available from satellites and is able to probe the different layers of cloud.
Long-term monitoring is essential to try and establish longer term changes in large-scale cloud systems – for this we must stitch different satellite instrument datasets together carefully and sensitively.
Even if we could represent cloud formation and evolution processes exactly in climate models – with the help of observations – clouds would still be a challenge due to their sensitivity to changes in the surrounding air mass – themselves influenced by changes in atmospheric and oceanic circulations and radiative heating.
In fact, as the Intergovernmental Panel on Climate Change’s fifth assessment report summarises (pdf), recent research suggests that many of the cloud changes that are projected for warmer climates are actually due to these large-scale circulation changes – and are less sensitive to the details of the small-scale cloud processes that we approximate.
These changes include a rise in altitude of high clouds, a decrease in mid- and high-level cloud cover, cloudy storm tracks shifting northwards in mid-latitudes, and decreases in the amount of low-level cloud.
The overall effect of these cloud changes is expected to bring further warming of the Earth’s surface, most likely causing a positive feedback loop that reinforces the warming caused by a strengthened greenhouse effect.
However, we can’t be absolutely certain because different global models from different modelling centres around the globe don’t all project the same changes in circulation pattern, and there are different approximations used to represent the cloud processes themselves.
Intriguing and challenging
Climate scientists have come a long way when it comes to understanding and modelling clouds, but there’s progress to be made.
So in summary, these are the things we know:
- Clouds cool the present day climate overall
- We are now able to represent cloud processes much better in at least some global climate models thanks to learning from much more detailed models – and doing this improves results
- Satellite observations of clouds have been helping to test our models
- Projections of future cloud changes vary due to approximations of cloud processes in the global models and differences in the future large scale circulation patterns between models.
And there are the things we could still do better:
- Many of the ways cloud at low altitudes form, evolve and dissolve are not clearly understood and models do not appear to represent them very well.
- The more detailed cloud resolving models need to be run over larger space and longer time domains to fully understand the benefits they bring
- Ice clouds have been less well studied and considered so far
- We need to maximise the information we can get from current and future satellite observations.
So it’s no wonder that clouds remain one of the most intriguing and challenging components of the climate system – and that’s why I have mixed feelings every time I fly through and above clouds. Even whilst I am appreciating their beauty, I have this infuriating feeling that there are still so many ways they can, and will, surprise us.